SESSION 9
LIQUID-LIQUID SEPARATION, PHENOMENA AND EQUIPMENT
Chairmen: S. Hartland, W. Zijl
COACERVATION CHARACTERISTICS OF NONIONIC SURFACTANTS AND THEIR APPLICATION TO METAL SEPARATION
Shigendo Akita*, Miquel Rovira**, Ana M. Sastre**, Nobuhisa Hyodo***, Hiroshi Takeuchi*** * Nagoya Municipal Industrial Research Institute, Nagoya, Japan ** Chemical Engineering Department, Universitat Politecnica de Catalunya, Barcelona, Spain *** Department of Chemical Engineeríng, Nagoya University, Nagoya, Japan
The aqueous solubility of a nonionic surfactant containing polyoxyethylene chain is dramatically
depressed above a well-defined temperature called as cloud point (CP). By allowing an aqueous
nonionic surfactant solution to settle at a temperature above the CP, the solution separates into two
homogeneous phases: one is rich in the surfactant concentration and the other is lean. This
phenomenon is known as coacervation of a nonionic surfactant. When a nonionic surfactant solution
containing solute is subject to phase-separation by heating, the solute may be partitioned between the
two phases, depending on its affinity to the surfactant. Thus, the two-phase system will provide a
separation field, and this novel separation method is referred to cloud point extraction. In previous
papers, we have studied the CP extraction of water-soluble aromatic compoundsl and gold(III)2.
The present study has been conducted to elucidate phase-separation (coacervation) characteristics of
nonionic surfactant solutions from viewpoints of both equilibrium and kinetics, including the
application of the aqueous two-phase system to metal separation.
Two polyoxyethylene nonyl phenyl ethers with an average chain length of 10 and 7.5 ethylene oxide
(PONPEl0 and PONPE7.5) were used as nonionic surfactant. An aqueous feed (20 cm3) containing
2.5 wt % surfactant was heated slowly until the onset of turbidity, which was noted as the CP of the
solution. Subsequently, the solution was allowed to be phase-separated by settling it overnight at a
prescribed settling temperature (ST).
Fig. 1 represents the effect of HCI concentration on the CPs of aqueous PONPE solutions. In all
cases, the CP rises with an increase in HCl concentration; this indicates that for higher HCI media
higher ST is required to attain phase-separation. The PONPE7.5 system gives much lower CP than
PONPEl0, owing to less hydrophilicity of the former surfactant. The addition of NaCl also gives
rise to a decrease in the CP. The phase-separation can be attained below the HCl concentration giving
CP lower than the prescribed ST, and the surfactant-rich phases were formed as the lower phase in all
the systems. It was found that the larger the temperature difference between CP and ST, the smaller
the equilibrium volume of the surfactant-rich phase becomes.
Fig. 2 shows a phase diagram of PONPEl0 solutions as a plot of the CP against the surfactant
concentration. We can get the composition of the two phases after phase-separation at a prescribed
ST, from the two intersections of the CP line parallel to the abscissa and the operating line as the ST.
The ST at the initial surfactant concentration (2.5 wt%) is regarded as a "plait point"; the loci on the
left and right sides of the point represent the compositions of the aqueous phase and of the surfactant-
rich phase, respectively. As the CP of the solution is far from the ST, the PONPEl0 concentration
tends to increase in the surfactant-rich phase, and conversely decrease in the aqueous phase.
Furthermore, the data for the system with NaCl can also be represented by the same binodal curve as
that without the salt, though giving rise to a CP decrease.
Fig. 3 shows typical phase-separation histories for the PONPEl0 system, as a plot of the height ratio
of the surfactant-rich phase to the whole liquid against the elapsed time, where the temperature
difference between ST and CP of the solution was induced by the addition of NaCl. The phase-
separation takes place relatively quickly even without centrifugation, then a volumetric equilibration is
attained within 10 min in all runs. It was found that there are two regimes in the history of
approaching the equilibrium height. For the temperature difference larger than ca. 10 oC, the height
of the surfactant-rich phase gradually increases, whereby an additional surfac,tant micelle piles onto
the layer formed previously in succession. For lower ST-CP, on the other hand, a larger surfactant-
rich phase is formed in early time, subsequently a slight decrease in the height occurs with time.
Fig. 4 shows typical results for the CP extraction of gold(III) and gallium(III) from HCl media.
Au(III) can be extracted from dilute HCl solutions; the PONPE7.5 system gives higher extraction
than the PONPEl0 system, and almost all the Au(III) is extracted in the surfactant-rich phase. A
decrease in the Au(III) extraction at higher HC1 concentrations may be attributable to a deterioration of
phase-separation due to a close value between the ST and CP. In contrast with Au(III), the Ga(III)
extraction is found to increase with increasing HC1 concentration.
It should be noted that these data were not obtained under optimized conditions, and hence there is a
possibility for getting more higher extraction efficiency by adjusting the parameters. Thus, CP
extraction might provide a novel method for recovery of unique metals.
REFERENCES
- Akita, S. and Takeuchi, H., Equilibrium Distribution of Aromatic Compounds between Aqueous Solution and Coacervate of Nonionic Surfactants, Sep. Sci. Technol., Vol.3 l, pp 401-412,1996.
- Akita, S. and Takeuchi, H., Cloud Point Extraction of Gold(III) from Hydrochloric Acid Solution, Proceeding of ISEC'96, Vol.1, pp 529-534,1996.

Fig.1 Effect of HC1 concentration on CP of aqueous PONPE solutions.

Fig.2 Binodal curves as relationship between CP and PONPEl0 concentrations in two phases.

Fig.3 Phase-separation history for PONPEl0 system.

Fig.4 Effect of HC1 concentration on CP extraction of Au(III) and Ga(III).
SEPARATION PROCESSES BY NON-DISPERSE LIQUID -LIQUID EXTRACTION MATHEMATICAL MODELLING OF THE SEPARATION SELECTIVITY
B.Galán, A.I. Alonso, M.F. San Román, A. Irabien, I.Ortiz Dpto. Química. Avda. los Castros s/n. 39005 Santander, Spain.
Non-disperse liquid-liquid extraction is a new technology widely use for the removal and/or
concentration of different metallic elements, anions, organic compounds, etc. In some cases the
objective of this process is not only the removal but the separation of the components of a
mixture; the separation of nickel and cadmium from highly concentrated solutions generated
during the leaching step of the recycling of Ni-Cd batteries is one example.
The right selection of the extractant agent is very important to obtain the individual separation
but usually it is not sufficient and in order to optimize the separation it is also necessary to
establish the operation conditions of the system. To set up the optimum operation conditions
of a separation process it is required to have a reliable design model of the system which
includes the selectivity of the4 components to be used as a reference parameter.
The experimental set up in a non-disperse system incorporates two hollow fiber modules and
three reservoir tanks where the phases are mixed homogeneously. The organic phase flows
from the extraction module to the back-extraction one; it is mixed in the organic phase
reservoir and enters again the extraction step. For the description of the mass transport
through this system, the macroscopic mass balance equations corresponding to the solutes
transport in the two hollow fiber modules must be combined with the equations describing the
change of solute concentration in the reservoirs.
The formulation of the mass balance equations for one solute have been described in the
literature (1,2). Recent works assume that the main resistance to the solute transport lies in the
membrane (3). When dealing with ionic species in the extraction step, it is also widely assumed
that chemical reactions are sufficiently fast to be considered as instantaneous, then the reacting
species are present in equilibrium concentration at the interface everywhere. Under these
assumptions, each reactive system is described by four partial differential equation (modules),
one algebraic equation (equilibrium condition) and three total differential equations
(tanks)(4,5). When two solutes are involved in the extraction and backextraction process, the
number of equations is duplicated. In order to establish the optimum operation conditions of
the separation process, equations describing the selectivity have to be introduced in the model.
The selectivity parameters in both modules, extraction and back extraction, depend on time
and position, local selectivity, .
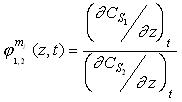
The feed and back-extraction tanks show a changing selectivity with time considered as
follows:
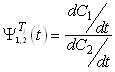
The integration of these equations in the module must be carried out with length (z) and time
(t) but in the tanks only time must be considered:
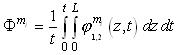
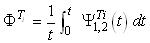
As a result, the system is described by eight partial differential equations (modules), two
algebraic equations (equilibrium constants), six total differential equations (tanks), four
differential equations and four integral equations (selectivity).
Although the complexity of the resultant model is high, it is important to remark that the
kinetic modelling reported in this work requires only the determination of four parameters: two
equilibrium parameters and two membrane mass transport coefficients.
The resolution of the equations taking as example the separation of a Ni-Cd mixture with
D2HEPA is carried out by the process modelling system, gPROMS.
BIBLIOGRAPHY
- M. I. Ortiz, B. Galán, A. Irabien, Kinetic analysis of the simultaneous non-dispersive extraction and back-extraction of Cr (VI). Ind. & Eng. Chem. Res., 35, pp 1369-1377, 1996.
- A.I. Alonso, C.C. Pantelides, Modelling and simulation of integrated membrane processes for recovery of Cr (VI) with Aliquat 336., J. of Memb. Sci., 110, pp 151-167, 1996.
- S.R. Wickramasinghe, M.J. Semmens and E.L., Cussler, Hollow fiber modules made with hollow fiber fabric, J. of Memb. Sci., 84, pp 1-14, 1993.
- M. I. Ortiz, B. Galán, A. Irabien., Membrane mass transport coefficient for the recovery of Cr (VI) in hollow fiber extraction and back-extraction modules. J. of Memb. Sci., 118, pp 213-221, 1996.
- A. Alonso, A.Urtiaga, S. Zamacona, A. Irabien and I. Ortiz., Kinetic modelling of Cd removal from phosporic acid by non-dispersive solvent extraction. J. of Memb. Sci., 130, pp 193-203,1997.
EXPERIMENTS ON PHASE INVERSION IN AQUEOUS TWO-PHASE SYSTEMS
J. C. Merchuk Dep. of Chemical Engineering and Unit of Biotechnology, Ben-Gurion University of the Negev, Beer Sheva, Israel.
ABSTRACT. Aqueous two-phase systems (ATPS) such as those formed by polyethylene
glycol (PEG) and dextran offer great potential for the separation of proteins in the presence
of other soluble materials, microbial cells and cell debris which can be difficult to separate
efficiently on a large scale. Most soluble and particulate material will partition to the
heavier, more polar phase and the target protein will partition to the lighter, less polar
phase (usually PEG). These systems have advantages over traditional adsorption
separations in their ability to process streams with small particulate material (whole broth
extraction) and to handle large volumes in a continuous mode.
In a large scale plant the possibility of using gravitational separation should always be
considered, because of the advantage in equipment initial inversion, mechanical simplicity,
maintenance and operation costs. This consideration is possible only if reliable basic
knowledge of the mechanism of phase separation is available. The present work is aimed at
establishing the background for the development of engineering methods in the design of
large scale gravitational separators for ATP systems.
Since the viscosity of the phases is generally very different, the kinetics of phase separation
is heavily influenced by which of the phases is continuos. Along a tie line, a point exist
where phase inversion occurs. Since the conductivity method used usually for monitoring
continuity of phases can not be used here, since both phases are aqueous, the best method
is the measurement of separation times.
Experimental data on the rate of separation of ATPS for the model system PEG/Phosphate
will be presented. From the changes of separation times the inversion point is obtained.
Consistency of the obtained results can be shown by extrapolation to zero tie line length.
The phase inversion point can be determined in the system studied (PEG 4000/Phosphate)
by a simple method based on measurement of separation times of samples taken along a tie
line. A range of ambiguity was found, where the continuity of the phases is affected not
only by the composition of the mixture, but also by the fluid dynamics. Within this range,
gentle agitation produces a bottom-continuous suspension, while strong agitation produces
a top-continuous suspension. Considering the large difference between the separation times
for each case, this finding is very relevant for the design of the separator in an ATPS.
DROP SIZE AND HOLD-UP PROFILES IN THE SEPARATION OF CRUDE OIL - WATER DISPERSIONS
K.Panoussopoulos, S.Hartland, P.E.Gramme*, T.Sentvedt*
Swiss Federal Institute of Technology ETH Dept. of Industrial and Engineering Chemistry CH-8092 Zurich Switzerland kostas@swix.ch
* NORSK HYDRO Petroleum Research Laboratory Research Centre P.O. Box 2560 N-3901 Porsgrunn, NORWAY
ABSTRACT
In this paper the separation of crude oil - water dispersions is investigated. New tools have been
developed to examine the variable behaviours of the liquid-liquid systems. A Gamma Density
Profile Apparatus scans with -rays inside an opaque mixture to obtain the concentration profiles
and especially the variation with time of the sedimenting and coalescing interfaces in batch settlers.
The position and the dynamic behaviour of the dense-packed region can be determined.
Additionally a newly developed Drop Video Monitoring system is presented that is able to record
on-line droplet sizes, also for dense-packed crude oil emulsions, and calculate their distributions.
The combination of the two tools explained several effects, like rapid-, slow separation, stable
emulsions. Laboratory experiments have been performed, with and without addition of chemicals,
to simulate and improve the situation in offshore oil fields in which the separation of the emulsions
should be accelerated.
The main task of the processing facilities in offshore oil and gas production is to separate the
gas-, oil- and water phases and treat the individual phases in order to satisfy product specifications.
Normally, the treated oil should not contain more than 0.5 % water and the produced water less than
40 ppm oil. The task of separating the oil/water dispersion is normally carried out in horizontal
gravity separators with the density difference as the driving force.
The cost of oil and gas production is strongly related to the physical size and weight of the
separation facilities. Furthermore the economical impact of the separation facilities increases with
the sea water depth at the site of production and with the diffculty of separating the oil and water.
Especially in case of heavier oils, the oil/water separability may be low due to the presence of
asphaltenic particles at the droplet interface and high oil viscosity counteracting water droplet
coalescence, which makes the droplet sedimentation velocity slower. To enhance separation,
chemical demulsifiers are normally used. In order to be able to design cost effective production
facilities it is vital to have available precise experimental techniques for characterising the oil/water
separability using fluid samples and further design methods that can predict the actual size of the
field separators on the basis of the laboratory data.
The separation of liquid-liquid dispersions can be studied in batch gravity settlers in which the
dispersion is calm and undisturbed. The crude oil, always being black in colour, makes the water
dispersions opaque and only the separated water phase is visible. With our novel Position Sensitive
Densiometer it is now possible to study rapidly sedimenting oil/water dispersions using gamma-rays
and to observe the variation of the hold-up of the dispersed phase with the height of the dispersion
and time. The Drop Video Monitoring system for dense-packed crude oil emulsions makes possible
to determine on-line the droplet sizes. Using such data it is possible to estimate the required full-
scale separator size using models developed based on sedimenting systems and the behaviour of
dense-packed droplet layers normally formed at the oil/water interface.
The Gamma Density Profile Apparatus 'GDPA'
Drop Video Monitoring system for dense-packed crude oil emulsions DVM
|